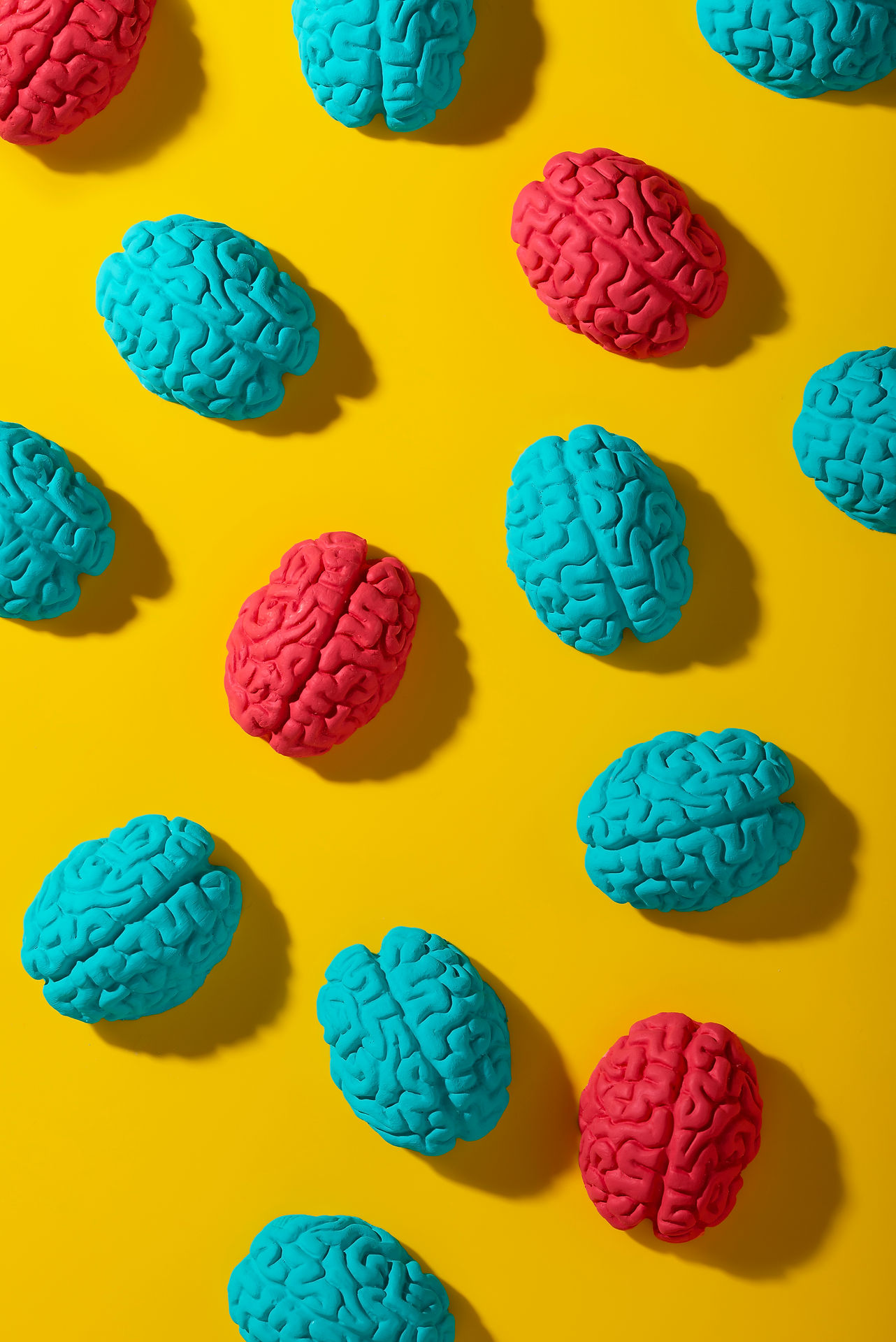
Ono Research Unit
Nagoya University, Research Institute of Environmental Medicine, Department of Neural Regulation
私たちは、時刻や光の情報が一切ない部屋で生活をしても、睡眠・覚醒や体温はおよそ24時間周期で変動します。このおよそ24時間のリズムを「概日リズム」と呼び、「概日時計」がその調節を担います。また、動物は季節(日長の変化)により、繁殖機能や生理機能を劇的に変化させ、厳しい環境を乗り越えています。つまり、1日のうち最適な時間に睡眠、繁殖、摂食を行う事で、動物は厳しい地球環境を乗り越えているわけです。しかし、これらの動物行動や生理機能のタイミングを調節する神経メカニズム、特に概日時計による調節メカニズムはよく分かっていません。私たちの研究チームは、動物が示す様々な行動や生理機能のタイミングを調節する、神経回路の動作原理の理解を目指しています。そのため、光操作や光イメージング、電気生理学、組織学、動物行動学に加え、ウイルスベクターやゲノム編集等の技術を総動員し、概日時計の中枢である視床下部に位置する「視交叉上核」を起点とした脳内神経ネットワークの全貌を明らかにしていきます。
研究は楽しいものです。子供のころのワクワクした感覚を今の研究に重ね合わせ、常に面白いと思うものを日々追求しています。研究をしたいと少しでも思った人はご連絡ください。研究への情熱さえあれば経験は問いません。
Animals anticipate changes in their environment and adjust their physiological functions accordingly. Many physiological functions, including sleep, wakefulness, and reproductive functions, are regulated by the circadian clock. In mammals, the central circadian clock is located in the suprachiasmatic nucleus (SCN) of the hypothalamus. Individual SCN neurons exhibit an oscillation of approximately 24 hours, which is referred to as the "circadian rhythm". Importantly, the circadian rhythms of individual SCN neurons are synchronized and exhibit robust circadian rhythms. This synchronization of circadian rhythms in the SCN is extremely important for the regulation of physiological functions.
The main goal of our research is to understand how the central circadian clock, the suprachiasmatic nucleus (SCN), regulates physiology and behavior. To understand the functional neural circuits that control the timing of behavior and physiology, such as sleep/wakefulness, body temperature, daily torpor, and hibernation, we use optical manipulation, optical imaging, electrophysiology, animal behavior, viral vectors, and genome editing methods to elucidate the entire brain neural network originating from the SCN. This research is critical to understanding how animals anticipate and adapt to predictable environmental cycles through their physiological functions.
We also focus on a novel circadian clock system that is generated in the absence of classical transcription-translation negative feedback loops (TTFLs) in mammals. The clock gene was thought to be an essential component of circadian rhythms. Previously, we found that circadian rhythms are still present in SCN neurons of Cry-deficient mice, which exhibit arrhythmic behavior (Ono et al., 2013, 2016). Therefore, we need to reconsider the classical TTFL model that is thought to constitute the circadian system and find a new circadian system without TTFL. We have established sophisticated techniques such as optical recording and manipulation at the single-cell level to identify the non-TTFL circadian clock. Since cyanobacteria have a non-TTFL circadian clock, I strongly believe that mammals also have a non-TTFL circadian clock system.
Research is fun. I overlap the excitement I felt as a child with my current research, and I always pursue things that I find interesting. If you are interested in our research, please let me know. Experience doesn't matter as long as you have a strong passion for research.
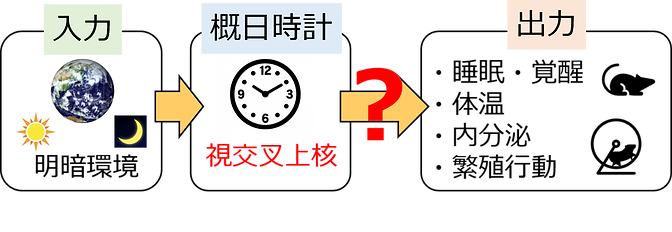
【研究テーマ】
1.行動・生理機能のタイミングを調節する神経回路の探索
動物は1日のうち睡眠をとりますが、睡眠中は無防備で外敵に襲われる可能性が高まります。したがって、いつどのタイミングで睡眠をとるかは動物にとって非常に重要な問題です。概日時計の中枢である視交叉上核を電気破壊すると、睡眠・覚醒のリズムが消失する事から、哺乳類において視交叉上核は、概日リズム調節に重要な神経細胞であると言えます。しかし、そこからどの神経回路を介して睡眠・覚醒のタイミングが調節されているかはよく分かっていません。
視交叉上核の神経発火の頻度は昼間に上昇し、夜間は低下するリズムを示しますが、この発火パターンは夜行性および昼行性動物で同じであることが分かっています。つまり、視交叉上核の概日リズム情報は、そこから先の下流神経回路で反転し、最終的な行動に出力されていると考えられています。このメカニズムも未だ明らかにされていません。さらに視交叉上核は、睡眠・覚醒以外にも体温リズムや内分泌リズム、繁殖機能、日内休眠、冬眠など様々な生理機能を調節していまることも分かっています。
私たちは、これら複雑な生理機能のタイミングを調節する神経回路を明らかにすることを目指しています。その目的の為、光遺伝学を用いて特定細胞の活動を調節し、光イメージングを用いて特定細胞の活動をリアルタイムに計測し、in vivoゲノム編集を用い特定機能を操作する事でそのメカニズムに迫ります。これまで、視交叉上核から複数の出力経路の同定および、そのうちの一つが睡眠・覚醒のタイミングを調節する事を明らかにしてきました (Ono et al., 2019 Communication Biology; Ono et al., 2020 Science Advances)。遺伝子改変マウスと光操作、光計測技術を用い、視交叉上核を起点とする新しい神経回路および関連分子の同定を進めています。
1. Sleep and wake regulation and circadian physiology in mice
The role of the brain’s central clock in maintaining time is well characterized, but how the clock communicates temporal information to physiology and behavior is still poorly understood. One fundamental output behavior is the cycle of sleep and wakefulness. To identify the underlying neuronal mechanisms that mediate circadian-regulated sleep and wakefulness, we introduced optogenetics, pharmacogenetics, in vivo fiver photometry, neuronal tracing, and sleep recordings, and for the first time, identified neuronal pathways in the hypothalamus that connect SCN rhythmic function to mammalian sleep and wakefulness (Ono et al., 2019, 2020). We also showed that the interaction of orexin and MCH peptides in the lateral hypothalamus induced severe sleep attacks (Hung and Ono et al., 2020). More recently, we have focused on how the SCN regulates daily torpor or hibernation to investigate the relationship between the circadian clock and metabolism.
-
*Ono, D., Mukai, Y., Hung, J.C., Chowdhury, S., Sugiyama, T., and *Yamanaka, A. The mammalian circadian pacemaker regulates wakefulness via CRF neurons in the paraventricular nucleus of the hypothalamus. Science Advances 6(45), 10.1126/sciadv.abd0384 (2020) (*Corresponding authors).
-
#Hung, J.C., #Ono, D., Kilduff, S.T., and Yamanaka, A. Dual Orexin and MCH neuron-ablated mice display severe sleep attacks and cataplexy. eLife 9:e54275, doi: 10.7554/eLife.54275 (2020) (# Co-first author).
-
*Ono, D., Honma, K., Yanagawa, Y., Yamanaka, A., and *Honma, S. GABA in the suprachiasmatic nucleus refines circadian output rhythms in mice. Commun. Biol. doi: https://doi.org/10.1038/s42003-019-0483-6 (2019) (*Corresponding authors).
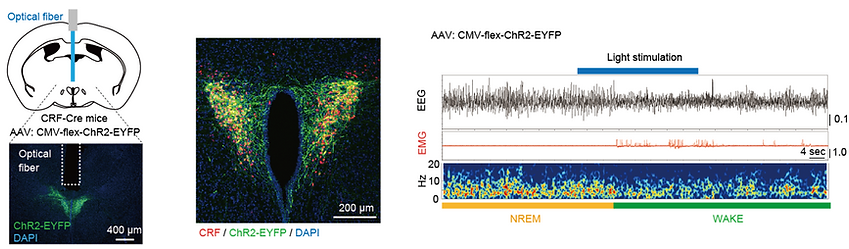

室傍核CRF神経にChR2を発現させ、光照射により神経活動を活性化する事で覚醒時間が増加する
2.概日時計の神経ネットワーク機構の解明
視交叉上核の個々の細胞は、時計遺伝子の転写翻訳を介したネガティブフィードバックループにより24時間の自律的なリズムが生じています。視交叉上核の細胞時計の周期や頑健性は、個々の細胞ごとに異なりますが、神経ネットワークを介する事で、同期し調和のとれたリズムが形成されています。神経回路は生後に劇的な変化を示し、発達過程における内因・外因性の要因によりその性質が左右されます。しかし、どの分子が概日時計の神経ネットワークの生後発達に関連しているのかはよく分かっていません。最近私たちは、時計遺伝子の一つであるCryptochrome (Cry) が、視交叉上核の神経ネットワークの生後発達に関連する事を明らかにしました (Ono et al., 2013 Nature Communications; Ono et al., 2016 Science Advances)。さらに、時計遺伝子Cryが概日時計の自律振動に必須でないことも明らかにしました。新しい光操作・光計測ツールの開発を行い、概日時計の神経ネットワークの可塑性に着目し研究を進めています。
2. Circadian regulation of cellular rhythms and neuronal network in the central circadian clock
Circadian rhythms are believed to be generated by a transcription-translation negative feedback loop (TTFL) involving several clock genes and their protein products. Among them, cryptochrome (Cry) has been thought to be an essential clock gene in mammals, because circadian behavior is arrhythmic in Cry1/Cry2 deficient mice under constant conditions. However, using bioluminescence or fluorescence imaging of the central clock in the suprachiasmatic nucleus (SCN), we discovered that individual neurons remained rhythmic in these behaviorally arrhythmic mice. This finding points to TTFL-independent oscillatory mechanisms in SCN neurons in mammals (Ono et al., 2013; Enoki and Ono et al., 2017; Ono et al., 2021). This has led me to search for new circadian clock mechanisms.
We also identified a novel role for mammalian Cry genes. Cry’s role outside of clock function was not known, though it serves multiple functions in other species. For instance, Cry is involved in plant photoreception and fly magnetoreception. We have found that mammalian Cry has a role in the maturation of neuronal networks in the SCN (Ono et al., 2016). We also found that during development, Cry normally supports the maturation of the neural network through arginine vasopressin and vasoactive intestinal peptide signaling. These findings yielded significant insight into underlying mechanisms of plasticity of neuronal networks in the central circadian clock in mammals.
-
Ono, D., Honma, K., Schmal, C., Takumi, T., Kawamoto, T., Fujimoto, K., Kato, Y., and Honma, S.: CHRONO and DEC1/DEC2 compensate for lack of CRY1/CRY2 in expression of coherent circadian rhythm but not in generation of circadian oscillation in the neonatal mouse SCN. Scientific Reports (2021).
-
#Enoki, R., #Ono, D., Kuroda, S., Honma, S., and Honma, K.: Dual origins of the intracellular circadian calcium rhythm in the suprachiasmatic nucleus. Scientific Reports 7, 41733. doi: 10.1038/srep41733. (2017) (# Co-first author).
-
Ono, D., Honma, S., and Honma, K.: Differential roles of AVP and VIP signaling in the postnatal changes of neural networks for coherent circadian rhythms in the SCN. Science Advances 2, e1600960 (2016).
-
Ono, D., Honma, S., and Honma, K.: Cryptochromes are critical for the development of coherent circadian rhythms in the mouse suprachiasmatic nucleus. Nature Communications 4:1666 doi: 10.1038/ncomms2670 (2013).
-
Ono, D.*, Wang, H., Hung, J.C., Wang, T.H., Kon, N., Yamanaka, A., Li, Y., and Sugiyama, T. Network-driven intracellular cAMP coordinates circadian rhythm in the suprachiasmatic nucleus. Science Advances 9(1), DOI: 10.1126/sciadv.abq7032 (2023).
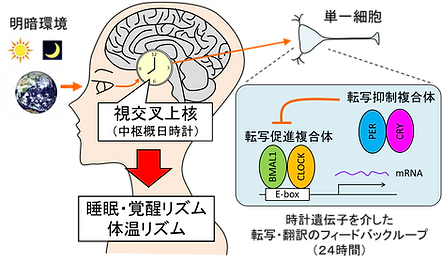
動画1:視交叉上核の分散培養
個々の細胞の概日時計は同調せず、周期や位相が異なるリズムがみられる。
動画2:視交叉上核のスライス培養
個々の細胞の概日時計は神経ネットワークを介し同調し、安定したリズムが形成されている。
3.光イメージング技術開発
これまでの生命科学分野では、「蛍光タンパク質」を用いた光イメージング技術により、細胞内カルシウム・cAMP・膜電位など、様々な細胞内イベントの計測が可能となってきました。一方で、光を用いて細胞機能を操作する「光遺伝学」により、細胞活性や機能を、高い時間・空間分解能で操作することが可能となり、大きな革命をもたらしています。しかしこれらの「操作」と「計測」には両者とも「光照射」が必要であり、可視光領域の波長の重複から、光操作・計測ツールの同時使用に大きな制限が生じています。もう一つの問題は生体深部からの細胞機能計測には、電極や光ファイバーを挿入しなければいけない点にあります。複雑な生理機能を理解する為には、神経回路を破壊することなく細胞機能を計測できる非侵襲的細胞機能計測が有効になると考えます。そのため、①生きた個体内の特定細胞の操作と計測を同時に行う技術、②非侵襲的な生体機能計測を行う技術、を開発する事が重要になります。これまで、発光タンパク質を用いたin vivo遺伝子発現計測の技術開発を進めてきました (Ono et al., 2015 Scientific Reports; Ono et al., 2017 PNAS)。これらの技術を応用し、励起光を必要としない新規発光タンパク質を用いたプローブ開発を進めています (Ono et al., 2020 Science Advances)。この新規光ツールを用い、生体深部からの細胞活動を非侵襲的に行うことが可能な近赤外発光イメージングの開発を進めています。
3. Development of optical imaging systems
In the life science field, fluorescence imaging and optogenetics have been widely used for recording and manipulation of cellular functions, respectively. But these powerful tools both require excitation light, so it is impossible to use the same wavelength to record and manipulate cellular function at the same time. To solve this problem, we established a new calcium bioluminescent probe, Okiluc-CaM, that requires no excitation, in order to measure and manipulate cellular function simultaneously (Ono et al., 2020). We also developed a simultaneous recording system for multiple cellular functions (Ono et al., 2017; Enoki and Ono et al., 2017). With these new methods, we identified novel aspects of neuronal networks in the SCN. For instance, we found that the clock genes Per1 and Bmal1 had independent oscillatory mechanisms in the SCN and that circadian calcium rhythms could still be detected in Cry deficient mice. We are now extending my focus to the functional roles of second messenger signaling on neuronal networks in the SCN.
Fluorescence proteins are often used to monitor cellular functions in vivo, but excitation light can produce autofluorescence and phototoxicity. Thus, long-term recording of cellular function using fluorescence is not easy to perform. To overcome these difficulties, we used a bioluminescence tool for in vivo research, and successfully measured clock gene expression from deep brain structures for more than a month in freely moving mice (Ono et al., 2015, 2017). Circadian rhythms in both central and peripheral tissues showed different phases between in vivo and ex vivo preparations, and episodic burst expression was observed in the SCN. These in vivo experiments provided significant insight into circadian rhythms at a system level.
-
*Ono, D., Mukai, Y., Hung, J.C., Chowdhury, S., Sugiyama, T., and *Yamanaka, A. The mammalian circadian pacemaker regulates wakefulness via CRF neurons in the paraventricular nucleus of the hypothalamus. Science Advances 6(45), 10.1126/sciadv.abd0384 (2020) (*Corresponding authors).
-
*Ono, D., *Honma, S., Nakajima, Y., Kuroda, S., Enoki, R., and Honma, K.: Dissociation of Per1 and Bmal1 circadian rhythms in the suprachiasmatic nucleus in parallel with behavioral outputs. Proc. Natl. Acad. Sci. U S A. doi: 10.1073/pnas.1613374114. (2017) (*Corresponding authors).
-
#Enoki, R., #Ono, D., Kuroda, S., Honma, S., and Honma, K.: Dual origins of the intracellular circadian calcium rhythm in the suprachiasmatic nucleus. Scientific Reports 7, 41733. doi: 10.1038/srep41733. (2017) (# Co-first author).
-
Ono, D., Honma, K., and Honma, S.: Circadian and ultradian rhythms of clock gene expression in the suprachiasmatic nucleus of freely moving mice. Scientific Reports 5, 12310; doi: 10.1038/srep12310 (2015).
-
Ono, D.*, Wang, H., Hung, J.C., Wang, T.H., Kon, N., Yamanaka, A., Li, Y., and Sugiyama, T. Network-driven intracellular cAMP coordinates circadian rhythm in the suprachiasmatic nucleus. Science Advances 9(1), DOI: 10.1126/sciadv.abq7032 (2023).
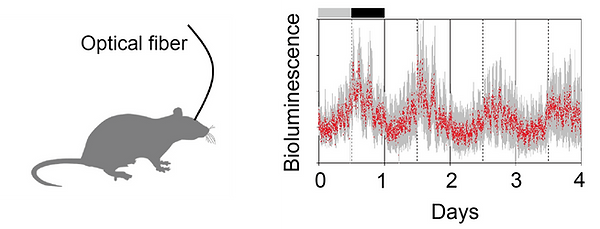